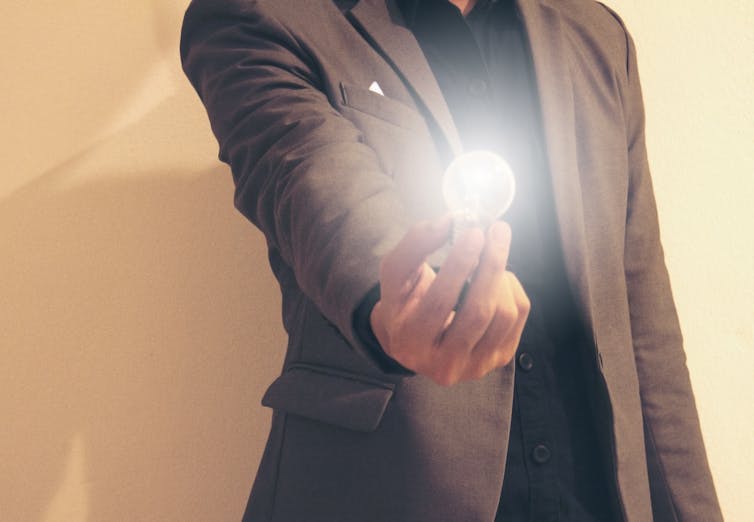
ellissharp/Shutterstock.com
Jalees Rehman, University of Illinois at Chicago
In a recent survey of over 1,500 scientists, more than 70 percent of them reported having been unable to reproduce other scientists’ findings at least once. Roughly half of the surveyed scientists ran into problems trying to reproduce their own results. No wonder people are talking about a “reproducibility crisis” in scientific research – an epidemic of studies that don’t hold up when run a second time.
Reproducibility of findings is a core foundation of science. If scientific results only hold true in some labs but not in others, then how can researchers feel confident about their discoveries? How can society put evidence-based policies into place if the evidence is unreliable?
Recognition of this “crisis” has prompted calls for reform. Researchers are feeling their way, experimenting with different practices meant to help distinguish solid science from irreproducible results. Some people are even starting to reevaluate how choices are made about what research actually gets tackled. Breaking innovative new ground is flashier than revisiting already published research. Does prioritizing novelty naturally lead to this point?
Incentivizing the wrong thing?
One solution to the reproducibility crisis could be simply to conduct lots of replication studies. For instance, the scientific journal eLife is participating in an initiative to validate and reproduce important recent findings in the field of cancer research. The first set of these “rerun” studies was recently released and yielded mixed results. The results of 2 out of 5 research studies were reproducible, one was not and two additional studies did not provide definitive answers.
There’s no need to restrict these sort of rerun studies to cancer research – reproducibility issues can be spotted across various fields of scientific research.
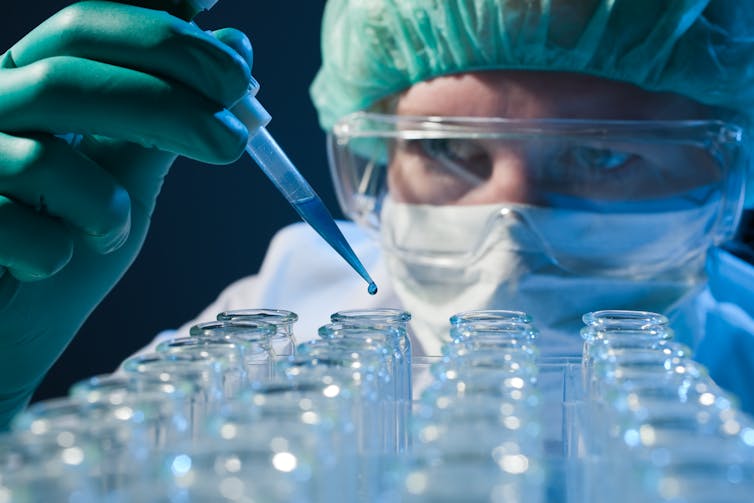
Alexander Raths/Shutterstock.com
But there’s at least one major obstacle to investing time and effort in this endeavor: the quest for novelty. The prestige of an academic journal depends at least partly on how often the research articles it publishes are cited. Thus, research journals often want to publish novel scientific findings which are more likely to be cited, not necessarily the results of newly rerun older research.
A study of clinical trials published in medical journals found the most prestigious journals prefer publishing studies considered highly novel and not necessarily those that have the most solid numbers backing up the claims. Funding agencies such as the National Institutes of Health ask scientists who review research grant applications to provide an “innovation” score in order to prioritize funding for the most innovative work. And scientists of course notice these tendencies – one study found the use of positive words like “novel,” “amazing,” “innovative” and “unprecedented” in paper abstracts and titles increased almost ninefold between 1974 and 2014.
Genetics researcher Barak Cohen at Washington University in St. Louis recently published a commentary analyzing this growing push for novelty. He suggests that progress in science depends on a delicate balance between novelty and checking the work of other scientists. When rewards such as funding of grants or publication in prestigious journals emphasize novelty at the expense of testing previously published results, science risks developing cracks in its foundation.
Houses of brick, mansions of straw
Cancer researcher William Kaelin Jr., a recipient of the 2016 Albert Lasker Award for Basic Medical Research, recently argued for fewer “mansions of straw” and more “houses of brick” in scientific publications.
One of his main concerns is that scientific papers now inflate their claims in order to emphasize their novelty and the relevance of biomedical research for clinical applications. By exchanging depth of research for breadth of claims, researchers may be at risk of compromising the robustness of the work. By claiming excessive novelty and impact, researchers may undermine its actual significance because they may fail to provide solid evidence for each claim.
Kaelin even suggests that some of his own work from the 1990s, which transformed cell biology research by discovering how cells can sense oxygen, may have struggled to get published today.
Prestigious journals often now demand complete scientific stories, from basic molecular mechanisms to proving their relevance in various animal models. Unexplained results or unanswered questions are seen as weaknesses. Instead of publishing one exciting novel finding that is robust, and which could spawn a new direction of research conducted by other groups, researchers now spend years gathering a whole string of findings with broad claims about novelty and impact.
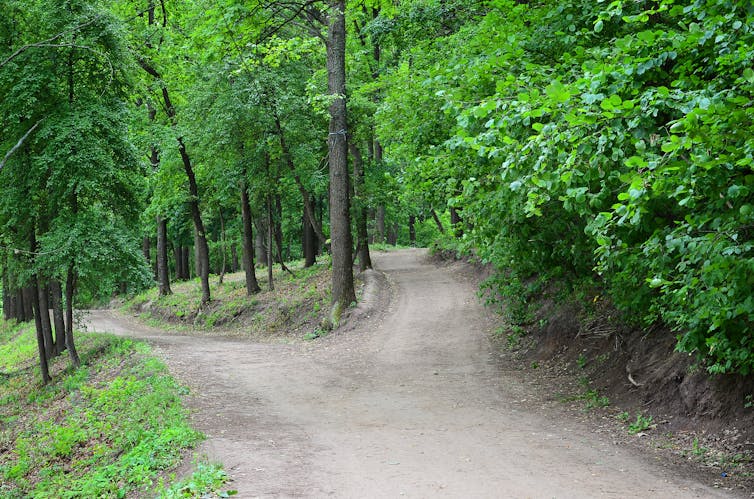
Mehaniq/Shutterstock.com
Balancing fresh findings and robustness
A challenge for editors and reviewers of scientific manuscripts is assessing the novelty and likely long-term impact of the work they’re assessing. The eventual importance of a new, unique scientific idea is sometimes difficult to recognize even by peers who are grounded in existing knowledge. Many basic research studies form the basis of future practical applications. One recent study found that of basic research articles that received at least one citation, 80 percent were eventually cited by a patent application. But it takes time for practical significance to come to light.
A collaborative team of economics researchers recently developed an unusual measure of scientific novelty by carefully studying the references of a paper. They ranked a scientific paper as more novel if it cited a diverse combination of journals. For example, a scientific article citing a botany journal, an economics journal and a physics journal would be considered very novel if no other article had cited this combination of varied references before.
This measure of novelty allowed them to identify papers which were more likely to be cited in the long run. But it took roughly four years for these novel papers to start showing their greater impact. One may disagree with this particular indicator of novelty, but the study makes an important point: It takes time to recognize the full impact of novel findings.
Realizing how difficult it is to assess novelty should give funding agencies, journal editors and scientists pause. Progress in science depends on new discoveries and following unexplored paths – but solid, reproducible research requires an equal emphasis on the robustness of the work. By restoring the balance between demands and rewards for novelty and robustness, science will achieve even greater progress.
Jalees Rehman, Associate Professor of Medicine and Pharmacology, University of Illinois at Chicago
This article was originally published on The Conversation. Read the original article.